Better batteries for the future
The lithium ion battery has become a core feature of daily life, working continuously inside our phones and laptops. But the global energy crisis has necessitated yet more ambitious uses for batteries. Electric vehicles are set to replace petrol and diesel cars, and beyond this, batteries also offer a solution to large scale grid energy storage. However, for a battery powered future to become a reality they must become cheaper, more sustainable and possess much greater energy densities. 40 years after the pioneering work of Nobel Prize winners M. Stanley Whittingham and John B. Goodenough in Oxford’s Inorganic Chemistry Laboratory, Oxford scientists are continuing to carry out fundamental research that will inform and shape the future of batteries.
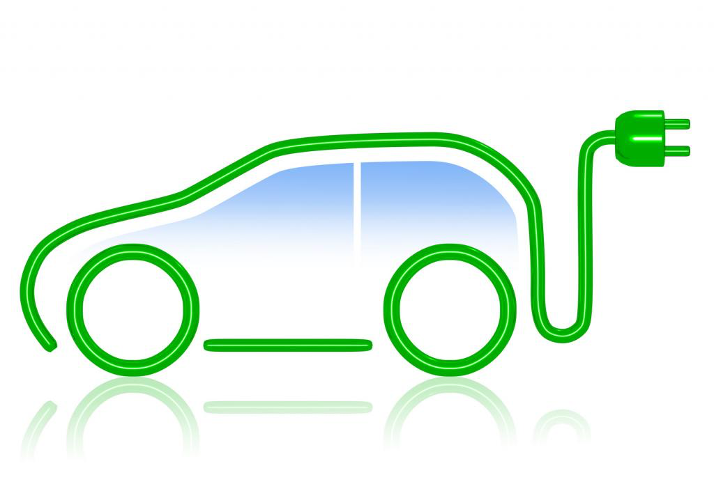
Much of the research taking place is funded by the Faraday Institution – a multimillion pound government initiative with the focus of developing batteries for the electric vehicle market. While there are many different avenues to improving battery technology, work in the Oxford Chemistry department is centred on two key areas: new materials for electrodes, and the development of solid-state batteries.
The FutureCat project is a collaboration between 5 UK universities and 9 industrial partners, led by Professor Serena Corr of the University of Sheffield. The overall aim is to improve the durability, power and sustainability of battery cathodes. At Oxford, the Clarke, Hayward and Goodwin groups are targeting the making and understanding of new cathode materials.
The cathodes used in most commercial lithium-ion batteries are variations on LiCoO2 based materials, in which the cobalt is reduced during discharge and oxidised on charging, cycling between +4 and +3. Cobalt presents a number of major problems, both ethical and economic. Much of the world’s cobalt reserves are in the Democratic Republic of Congo. The mining industry in this country is rife with exploitation, with child labour and modern slavery widespread in the dangerous and unregulated cobalt mines. Indeed, Apple, Tesla and Microsoft were recently sued over their involvement in cobalt production in the DRC.
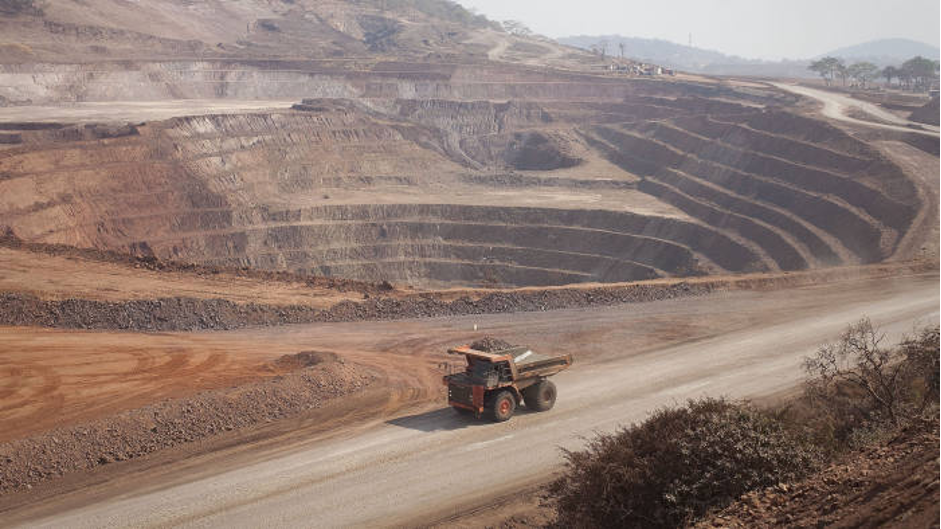
It is clear that cobalt mined in this way cannot sustainably be produced on a large enough scale to meet market demands. In an effort to solve this problem, battery chemists are trying to reduce or replace cobalt in cathode materials. This is the area that the groups of Simon Clarke and Michael Hayward group are working on. They are focussing on synthesis of completely new materials containing other transition metals, notably iron and manganese. Iron is of particular interest because of its high natural abundance and low toxicity. However, it is not a case of simply using LiFeO2 or LiMnO2, as these have different structures to LiCoO2 when prepared using conventional synthesis methods. Iron based materials also suffer from structural changes on cycling. Therefore, it is a great challenge to reduce the cobalt content while maintaining or improving energy density. Postdoctoral researchers Viktoria Falkowski and Xabier Martinez De Irujo Labalde are creating brand new metal oxides and oxysulfides, to provide new cathode materials and also to understand how they cycle. They are making use of the low temperature ‘topological’ synthesis methods that the Clarke and Hayward groups specialise in, rather than conventional high temperature synthesis. By using lower temperatures, metastable phases can be accessed, with very different structures and properties to the most thermodynamically stable material. This allows them to build their array of novel and interesting metal chalcogenides.
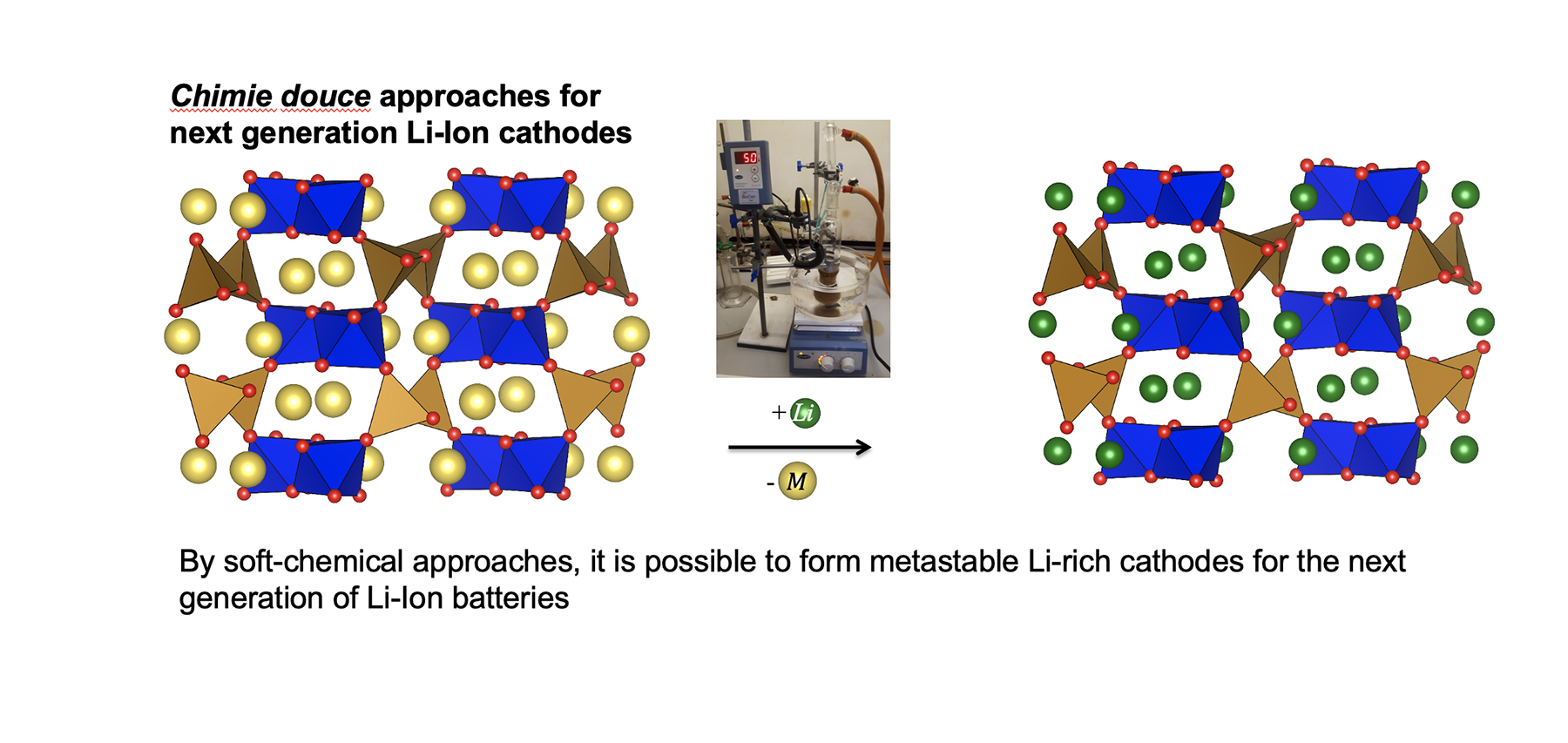
Essential to the development of new cathode materials is understanding the structural changes occurring within the materials throughout the battery charge and discharge cycle. The Goodwin group study these changes to help assess the suitability of materials. A recent article published in Nature Communications, in collaboration with scientists from The University of Cambridge, studies the charge reaction thermodynamics of Fe and Mn oxides in their reactions with lithium. The group uses X-ray Pair Distribution Function analysis, which is a synchrotron X-ray diffraction method that is particularly useful for probing materials possessing short-range order, rather than long range crystallinity. These methods allow the process to be studied in operando during cycling, rather than simply looking at the start and end states. Postdoctoral researcher in the Goodwin group, Harry Geddes, notes that in order to design materials with high performance, it is important to first understand their structure and properties.
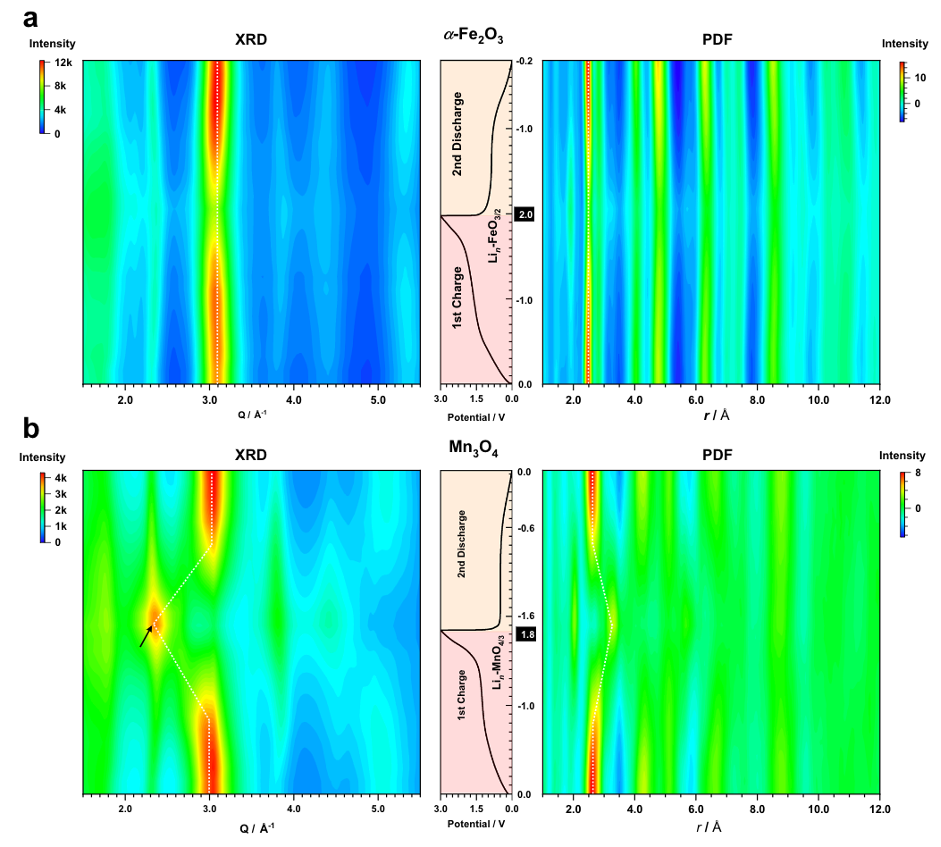
Figure: Contour plots from x-ray total scattering experiments performed on Fe2O3 and Mn2O3 during charge and discharge.
Another Faraday Institute initiative is the SOLBAT project, which aims to develop all-solid-state next generation batteries. This is desirable because of safety problems with organic solvent electrolytes, which can catch fire if a fault occurs as well as offering enhancements in battery performance such as lifetime.
The group of Charlotte Williams are targeting the interface between the cathode and the solid-state electrolyte. For lithium ions and electrons to move during battery operation, the active cathode and solid electrolyte particles must maintain physical contact. However, cathode particles undergo volume changes as lithium is taken up and removed requiring unpractical high pressures to be applied to maintain contact. A possible solution to this is to use a polymer binder or coating to accommodate the volume changes. In addition to having the right mechanical elastic response the polymer should also be ionically conductive so as not to impede the movement of Li-ions. Currently, polyethylene glycol has found use as an electrolyte, due to the ability of Li+ ions to hop along the oxygen atoms in its chains. However, its mechanical properties are poor being either a waxy liquid at low molecular weight or a brittle powder at high molecular weight.
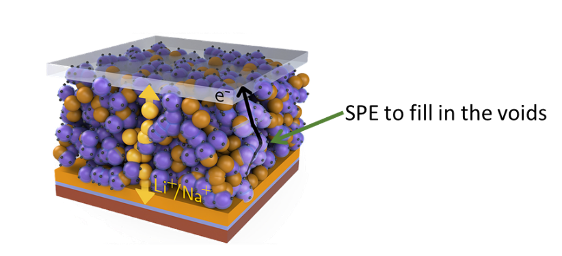
The Williams group have harnessed their expertise in synthesising polymers consisting of polyesters, polyethers and polycarbonates. The polycarbonate family has proven to be particularly suitable and yet more pleasingly, can be synthesised from carbon dioxide. The use of CO2 in these polymers has marked sustainability benefits, giving rise to a triple win in carbon dioxide emissions – for every molecule of carbon dioxide taken up into the polymer, two more are saved by avoiding the use of the epoxide. Through years of previous work, they have perfected controlled synthesis of such polymers, which is scalable, robust and reproducible. Postdoctoral researcher Georgina Gregory is working on building a systematic series of polymers, using well-refined and precise methods. She then works closely with members of the Bruce and Pasta groups in Oxford Materials, testing these polymers to determine their conductivity and electrochemical stability. The mechanical properties are also studied, to ensure the material adheres to the cathode and is able to accommodate the volume changes. Professor Williams emphasise that key to delivering the high performance solid state battery is close interdisciplinary collaboration between researchers working in Chemistry, Materials and Engineering.
It is clear that the wealth of knowledge within the Oxford Chemistry department in diverse areas of solid-state synthesis, computational methods and polymer chemistry are making an exciting contribution to the field of battery technology.
Article by Caitilin McManus
For more information, see:
Cathode material characterisation:
Hua, X., Eggeman, A.S., Castillo-Martínez, E. et al. Revisiting metal fluorides as lithium-ion battery cathodes. Nat. Mater. (2021).
Hua, X., Allan, P.K., Gong, C. et al. Non-equilibrium metal oxides via reconversion chemistry in lithium-ion batteries. Nat Commun 12, 561 (2021).
Polymer chemistry:
G. S. Sulley et al. Switchable Catalysis Improves the Properties of CO2-Derived Polymers: Poly(cyclohexene carbonate-b-ε-decalactone-b-cyclohexene carbonate) Adhesives, Elastomers, and Toughened Plastics. J. Am. Chem. Soc. 2020, 142, 9, 4367–4378
G. L. Gregory et al. Triblock polyester thermoplastic elastomers with semi-aromatic polymer end blocks by ring-opening copolymerization. Chem. Sci., 2020,11, 6567-6581
Faraday Project